Harvesting
Chemical Energy
- Cellular
Respiration -
Overview
Diagrams Review
Questions
Why do we eat? Why
do we breathe? Why do cells need energy? How do cells gain access to energy?
These are some of the questions which you can ponder as we move through
this section of the cellular world. Cells have the machinery to manipulate
molecules and the bonds within them. As covalent bonds are rearranged energy
is released. This energy is harvested by different means in different cells.
The goal is to replenish the ever dwindling supply of ATP which is necessary
to perform "work" in the cells. Most cells have a biochemical pathway referred
to as cellular respiration.
A
simple overview (but great) animation of cellular respiration
Harvesting
Chemical Energy
In this section it is important
to focus on the metabolic process, its purpose and how the extraction of
energy is accomplished. I have added some additional information in this
study guide to allow you to understand the process better.
Metabolic pathways:
Role
of enzymes. Each step in the pathways is catalyzed by a specific enzyme.
Catabolic versus anabolic
pathways
-
Catabolic reaction harvest energy
as well as building blocks by breaking down molecules. Energy is needed
for different forms of work, e.g. anabolic reactions, transport processes,
movement.
-
Anabolic reactions use the energy
and the building blocks to make molecules.
Energy carriers:
The cell has numerous molecules which are able to �hold� energy. The most
common type is adenosine triphosphate (ATP) which is used in most processes
which require energy within the cell. Other examples are NADH and FADH2
which carry substantially more energy than ATP. As you know the energy
in the latter two molecules is transferred to ATP in the electron transport
chain.
Release of energy:
Evolution has led to a number of metabolic pathways able to change the
structure of molecules. In the process of change the energy trapped in
the covalent bonds of the molecule is transferred to various other molecules
such as ATP, NADH and FADH2. This is all accomplished with the help of
specific enzymes. Recall that enzymes specifically fit their substrate
as they connect via the active site.
Cellular Respiration:
a process able to extract a large amount of energy from food molecules.
In eukaryotic cells oxygen is a required component. This metabolic process
is the main reason that animals have elaborate gas exchange organs such
as lungs, gills and other systems. The goal of these systems is, of course,
to get access to oxygen and to get rid of carbon dioxide.
C6H12O6 + 6 O2 + 6 H20
------- 6 C02 + 12 H20
ADP + P
ATP
Glucose + 6 Oxygen + 6 Water
----- 6 Carbon dioxide + 12 Water
ADP + P
ATP
The equation
above represents the input and output, i.e. the final outcome of the process.
As you can see the glucose molecule is completely oxidized to carbon dioxide
and water. Of course, to accomplish the complete breakdown of glucose it
is necessary to use numerous small steps. The glucose molecule contains
an enormous amount of energy and it is simply not possible to release all
the energy at once. Instead the numerous steps in the metabolic pathways
allows the energy to be captured in small increments as they are transferred
to ATP, NADH and FADH2.
Cellular respiration is composed
of three components:
-
i) Glycolysis
-
ii) Krebs Cycle (also called the
Citric Acid Cycle or the TCA Cycle)
-
iii) The Electron Transport Chain
(ETC)
Glycolysis:
The name of the pathway
refers to the breakdown (lysis) of glucose. Glycolysis is the most ancient
of all the metabolic pathways and is found in all organisms. It occurs
in the cytoplasm of the cell. In the process
of glycolysis the cell is able to break down one molecule of glucose
to two molecules of pyruvate,
Pyruvate
contains less energy than glucose. During the metabolic steps of glycolysis
energy is transferred from the molecular intermediates to energy carrying
molecules. 2 ATP and 2 NADH are formed from each molecule of glucose. The
point is that the amount of energy transferred only represents a minor
fraction of what is available in glucose. I.e. the pyruvate molecules contain
much more chemical energy if it can be accessed.
Some
bacterial species solely depend on the process as they do not contain the
following two pathways.
The Krebs Cycle:
These pathways
represent changes in organisms over evolutionary time making it possible
to extract further energy from the pyruvate molecules.
In
bacteria the Krebs Cycle occurs in the cytoplasm while in eukaryotic cells
it occurs in the matrix of the mitochondria. Consequently, the pyruvate
molecules (formed in the cytoplasm) need to be transported into the mitochondria.
The Krebs
cycle is preceded by a preparatory step in which pyruvate (a 3 carbon
molecule) is converted to Acetyl CoA (a 2 carbon molecule). In the process
energy is transferred to NADH. A carbon is released in the form of carbon
dioxide (CO2)
Acetyl
CoA enters a cyclic pathway by joining a four carbon molecule. This newly
formed molecule (Citric Acid �a six carbon molecule) now moves into a series
of metabolic steps catalyzed by different enzymes. As it is rearranged
energy as well as carbons (CO2) are released. Ultimately it will
join a new Acetyl CoA once again as the cyclic pathway continues.
All the
carbons in the molecule are ultimately turned into carbon dioxide as the
cycle keeps turning. The released energy is captured by ADP (producing
ATP) as well as NAD+ (producing NADH) and FADH (producing FADH2). In terms
of numbers each glucose molecule forms 6 CO2, 2 ATP, 8 NADH and 2 FADH2
in the process. As you can see glucose is completely oxidized to carbon
dioxide! A relatively small amount of ATP has been produced. In contrast
a large number of NADH has been formed. Recall that NADH holds more energy
than ATP molecules.
As
you are well aware cellular processes depend on the presence of ATP. The
dilemma at this point is that so far very little ATP has been produced,
The challenge is to convert the energy trapped in the NADH molecules to
a usable form, i.e. to somehow transfer the energy to ATP molecules.
The Electron Transport
Chain (ETC):
The
ETC also takes place in the mitochondria. A series of proteins are present
in the inner mitochondrial membrane (the cristae). The
electrons move through these proteins. Recall that membranes also serve
as selective barriers in a cell.
The
goal of the process is to transfer the energy contained in NADH to ATP
molecules. This is done in two steps:
-
i) A proton gradient is built
across the membrane. Such a gradient represents potential energy (an analogy
could be water on a hillside)
-
ii) As the protons move back through
the membrane the potential energy is used to make ATP molecules (an analogy
could be to allow the water on the hillside to fall and in the process
capture the energy via a turbine).
Building the Proton Gradient:
In
the first step the energy (in the form of an electron) is transferred from
NADH to a protein in the inner mitochondrial membrane. The NAD+ molecules
(now low in energy) return to the Krebs cycle to pick up more energy.
The transferred electrons travel from protein to protein in the mitochondrial
membrane. Ultimately the electron is transferred to an oxygen molecule
(the final electron acceptor) which combines with protons to form water.
As the electrons travel from protein to protein in the ETC they loose energy.
This energy is mostly lost as heat but some of it is used to transport
protons across the membrane. These protons are moved from the inside of
the mitochondria (matrix) to the inter-membrane space between the outer
and inner mitochondrial membrane. By this pumping action a proton gradient
is built across the membrane. The inter-membrane space has a high concentration
of protons while the matrix has a low concentration of protons.
Producing ATP:
As you know (based on your knowledge of diffusion) these molecules strive
towards returning across the membrane. Unfortunately (or perhaps fortunately),
the membrane does not allow these protons to cross the phospholipid bilayer.
The membrane also contains numerous proteins fulfilling different cellular
tasks. One of these proteins is ATP Synthase (also called ATPase). The
protons
can move across the membrane through the ATP synthase. As they do so
the potential energy contained in the gradient is transferred to ATP (ATP
forms from ADP and P), i.e. the energy from the NADH has now been transferred
to ATP. Of course, the energy had its initial origin in the covalent bonds
of a glucose molecule as it entered glycolysis.
The
newly formed ATP can now diffuse away from the mitochondria and perform
cellular work in other parts of the cell. As it is used up it is split
into ADP and P. These molecules now return to the mitochondria to �pick
up� energy from the electron transport chain. The supply of ATP is continuously
recycled.
An Overview
of Cellular Respiration
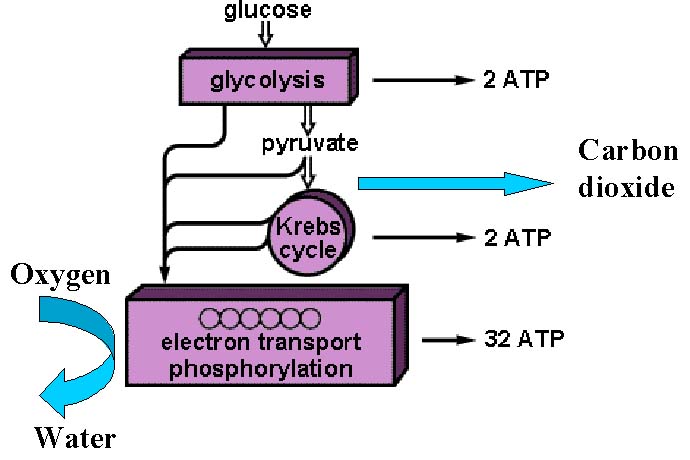
Chapter Review
Questions
-
Why do we breathe?
-
Is our "burning" of glucose an
efficient process?
-
Understand how energy contained
in chemical bonds can be transferred between molecules.
-
In this section you are introduced
to NADH. This molecule has the ability to "carry" a large amount of energy.
Remember how we referred to them in class as energy "shuttles".
-
Electron carriers and electron
transport chains refer to processes that can transfer electrons. These
electrons often end up on oxygen (thereby our great need for a constant
supply of oxygen). In the process the energy contained in the electrons
is transferred to other molecules (i.e. a different format that can be
utilized).
-
How to make ATP? One way is to
transfer part of the energy of a broken covalent bond of a "fuel" molecule
directly to ADP+P forming ATP. This is called Substrate-level phosphorylation.
The second way is to build a proton gradient across a membrane (remember
our water on the roof discussion?). As the protons fall back across the
membrane through an enzyme (ATPase) the call can make ATP (in our analogy:
as the water falls from the roof we can run a turbine).
-
Know the three stages of cellular
respiration: Glycolysis, the Krebs cycle and the electron transport chain
(ETC from now on). The first two yield a very small amount of ATP while
the last one yields a large amount of ATP.
-
Glycolysis: a biochemical pathway
shared by all cells on planet earth. Glucose is transformed to pyruvate
in the cytoplasm. In the process a small amount of ATP is formed at the
same time as some energy is transferred to NADH. The bulk of the energy
available is still contained within the pyruvate molecules. [Take
a look at the whole process in this
animation of glycolysis to get a feeling for the dynamic rearrangement
of the molecules as glucose is transformed to pyruvate. No need to memorize
the details of the pathway in the animation.]
-
As pyruvate enters the mitochondria
in eukaryotic cells it is transformed into acetyl CoA. In the process some
energy is transferred to NADH and a carbon is broken of the molecule. This
is the first example of how CO2 is forming within the cell.
-
The Krebs cycle occurs inside
the matrix of the mitochondria. Acetyl CoA is fed into a cyclic pathway.
The molecules are rearranged as they cycle around in the pathway. [Look
at the pathway in this
animated presentation of the Krebs Cycle. Notice the rearrangement
of the molecules (no need to memorize those details in our class).
I included the link so you can get a feeling for what metabolism is all
about (not to freak you out)]. In the process energy is transferred to
ATP as well as to NADH (and FADH2 which is a similar molecule as NADH).
Carbons are also released as CO2. At this point the glucose molecule has
literally been broken down to carbon dioxide. Most of the energy has been
released as heat but some energy has been transferred to ATP. The bulk
of the energy has been transferred to NADH (remember our $100 analogy?).
The goal now is to access this energy.
-
The energy contained in NADH is
used to produce ATP. The energy rich electrons from NADH is transferred
to a series of proteins in a membrane (the electron transport chain). The
electrons move through these proteins. As they move the electrons lose
energy and finally ends up on oxygen. The energy is used to move protons
across the membrane to build a proton gradient. This is a form of potential
energy (remember our "carry water up on the roof" analogy?). These protons
strive to return to the other side of the membrane but they cannot pass
the selective barrier of the membrane without "help". An enzyme called
ATP
synthase allows the protons to move through but take advantage of the
steady movement of these protons to produce ATP (similar to our water turbine
analogy in class). What would happen if oxygen was absent?
-
Different poisons can block the
movement of electrons in the transport chain.
-
Study the diagram above as you
try to connect the three processes discussed in this chapter into a view
of cellular respiration. What is accomplished? What is needed for the process
to occur? What is produced?
-
Fermentation: Understand the need
for oxygen in cellular respiration. If it is absent the cell needs to replenish
the precious NAD+ molecules to allow glycolysis to continue. (Recall our
bus company analogy). The energy contained in NADH is "dumped" to produce
another molecule so NAD+ can return to glycolysis. Thereby glycolysis can
proceed with a low yield of ATP. The "other molecule" is often referred
to as a fermentation product. Some examples are ethanol in yeast cells
as well as lactic acid in muscle cells (heavy exercise).
-
Understand how the building blocks
of all the macromolecules in food feed into different parts of the three
pathways discussed. Cellular respiration is kind of a major metabolic "freeway",
i.e. other expressways are linked to the main route.
-
In the same manner these molecules
can be "pulled out" of the pathway (kind of like exits on the freeway)
to provide building blocks for macromolecules in the anabolic pathways
of the cell.
-
It is important to understand
that the energy released and transferred in cellular respiration has its
origin in the process of photosynthesis (and, of course, originally in
the sun's energy).
Links: